[ad_1]
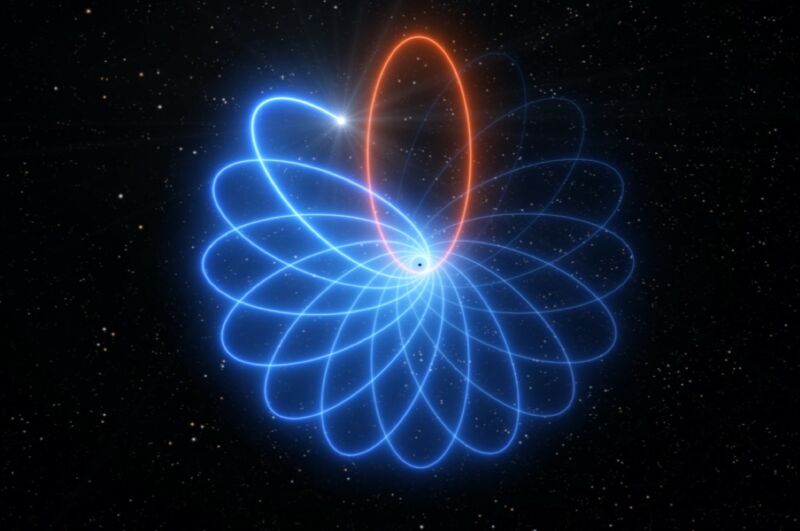
Almost 30 years have passed, but scientists in collaboration with the Very Large Telescope (VLT) in the Atacama Desert in Chile have now measured, for the first time, the unique orbit of a star orbiting the supermassive black hole believed to be lies at the center of our galaxy, the Milky Way. The star path (known as S2) traces a distinctive rosette-shaped pattern (similar to a spirograph), according to one of the central predictions of Albert Einstein’s general theory of relativity. The international collaboration described its results in a new article in the journal Astronomy and Astrophysics.
“General relativity predicts that the laced orbits of one object around another are not closed, as in Newtonian gravity, but are moving forward in the plane of motion,” said Reinhard Genzel, director of the Max Planck Institute for Extraterrestrial Physics ( MPE) in Garching, Germany. “This famous effect, first seen in the orbit of the planet Mercury around the Sun, was the first evidence in favor of general relativity. One hundred years later, we have detected the same effect on the motion of a star orbiting the compact Sagittarius A * (SagA *) radio source in the center of the Milky Way. “
When Einstein developed his general theory of relativity, he proposed three classical tests to confirm its validity. One was the deflection of light by the sun. Since massive objects deform and curve spacetime, light will follow a curved path around massive objects. This prediction was confirmed in 1919 with that year’s solar eclipse, thanks to Sir Arthur Eddington’s expedition to measure the gravitational deflection of starlight passing near the Sun. The confirmation made headlines around the world, and Einstein became in a familiar name.
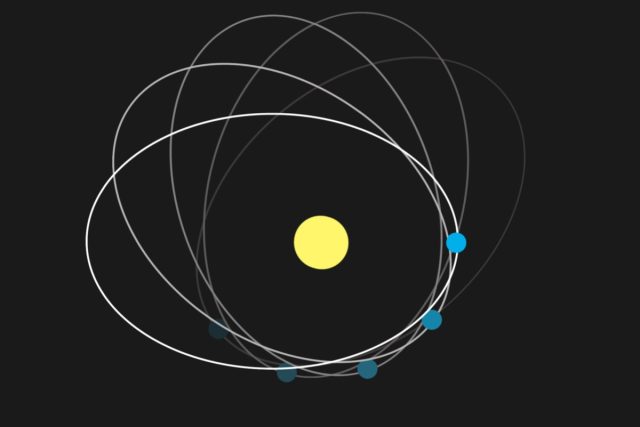
Rainer Zenz / Wikimedia Commons
General relativity also predicted a gravitational redshift of light in the presence of strong gravitational fields. That was first confirmed by measuring a redshift under the light of a white dwarf star in 1954.
The third test was the precession of Mercury’s rather eccentric orbit around the Sun. Every 100 years or so, the planet’s perihelion, or the point where it is closest to the Sun, is displaced by approximately 0.001 degrees, thanks to the attraction gravitational from other planets. That effect is how astronomers finally discovered Neptune. Astronomers had noticed some strange disturbances in Uranus’ orbit, and 19th century French mathematician Urbain Le Verrier correctly deduced that it was evidence from another planet; His prediction of 1845 was confirmed by observation in September 1846.
Le Verrier also attempted to model Mercury’s orbit according to Newtonian gravity, which was tested during Mercury’s transit in 1843. His model failed that test, and suggested that, once again, the deviations could be due to a planet. hypothetical not yet discovered even closer to the Sun, later called Vulcan. But in the following decades, there were no confirmed observations of such a planet. It was Einstein who demonstrated that Newton’s theory of gravity was incomplete. General relativity explains precisely the observed precession of Mercury’s orbit.

ESO / L. Calçada / spaceengine.org
If those key predictions of general relativity have already been confirmed experimentally, why are scientists so keen to continue testing them? Well, there may be unique environments beyond our solar system, for example, the extreme gravity of a supermassive black hole, where the laws of physics might not be the same. SagA * is the perfect laboratory to study this, especially given the dense cluster of stars that orbit around it. One of those stars, S2, is of particular interest, as it gets pretty close to the black hole during its closest approach (less than 20 billion km).
Enter the people behind the VLT, which first went online in 1998. The VLT team was able to detect the faint glow around the black hole when S2 passed in its first observations of the star. Approximately two years later, in 2018, they successfully measured S2’s gravitational redshift, so the strong gravity of the black hole stretches the star’s light to longer wavelengths as it passes. Infrared observations using the VLT’s GRAVITY, SYMPHONY, and NACO instruments showed that the amount of displaced light precisely matched the predictions of general relativity.
Like the redshift effect, the precession of S2’s orbit is small, which means it requires longer observation times before astronomers can detect them. S2 completes an orbit once every 16 years. The team finally gathered enough data points on the star’s position and speed, more than 330 measurements in all, to accurately map its orbit. And just as general relativity predicts, every time S2 passes near the supermassive black hole, it receives a gravitational “kick”, changing its orbit very slightly, so the orbital path forms that pretty rosette shape.
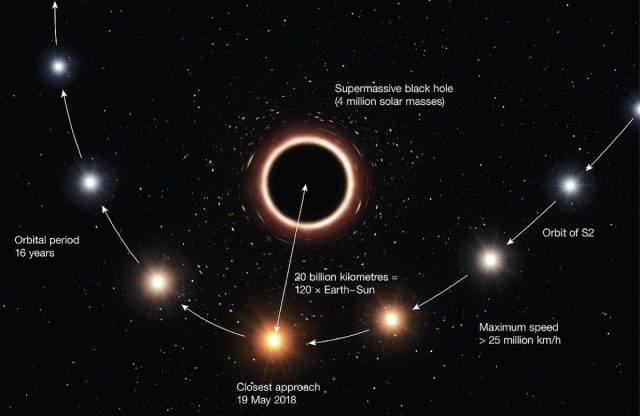
ESO / M. Kornmesser
“Our previous result has shown that the light emitted by the star experiences general relativity. Now we have shown that the star itself feels the effects of general relativity,” said Paulo García, from Portugal’s Center for Astrophysics and Gravitation, one of the GRAVITY leading scientists.
The next phase will be based on the next extremely large Telescope, which should give scientists the ability to see much fainter stars near the supermassive black hole. “If we are lucky, we could capture stars close enough to actually feel the rotation, the spin, of the black hole,” said Andreas Eckart of the University of Cologne, another project lead scientist, allowing astronomers to measure the SagA * twist and mass, as well as define the space and time around it. “That would be a completely different level of relativity testing again.”
DOI: Astronomy and Astrophysics, 2020. 10.1051 / 0004-6361 / 202037813 (About DOI).