[ad_1]
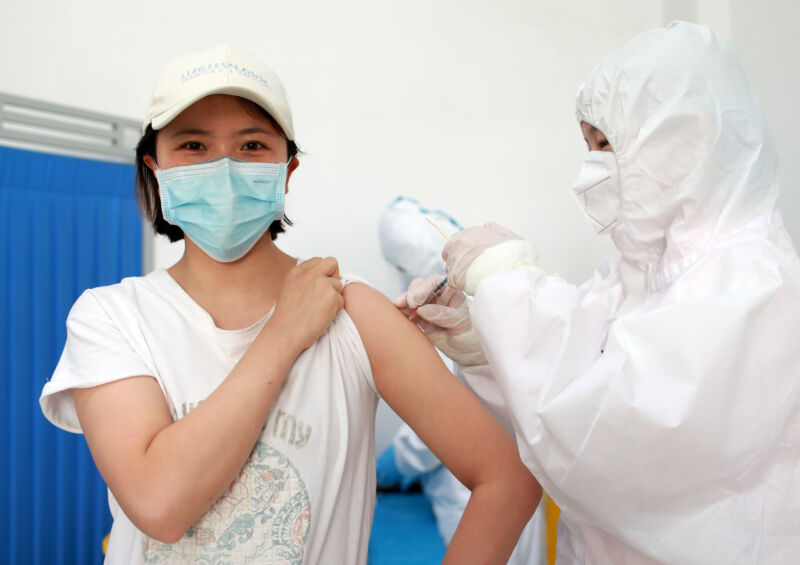
The clearest way out COVID-19’s crisis is developing a safe and effective vaccine, and scientists have wasted no time getting started.
They have at least 102 candidate vaccines in development worldwide. Eight of them have already entered the first clinical trials in people. At least two have protected a small number of monkeys from infection with the new coronavirus, SARS-CoV-2, which causes COVID-19.
Some optimistic vaccine developers say that if all goes well, we could see large-scale production and limited vaccine deployment as early as this fall. If true, it would be an extraordinary achievement. Less than four months ago, SARS-CoV-2 was an unnamed and never-before-seen virus that abruptly emerged in the central Chinese city of Wuhan. Researchers there quickly identified it and by the end of January they had cracked and shared its genetic code, allowing researchers around the world to work to defeat it. In late February, researchers from multiple continents were developing clinical trials for vaccine candidates. In mid-March, two of them started and the volunteers began to receive the first blows of candidate vaccines against COVID-19.
It is a record feat. But, it is not clear whether researchers will be able to maintain this breakneck pace.
In general, vaccines must go through three increasingly stringent human testing phases before they are considered safe and effective. The phases assess the safety profile of the candidates, the strength of the immune responses they trigger, and how good they are at protecting people from infection and disease.
Most vaccine candidates do not. By some estimates, more than 90 percent fail. And, while a pandemic-driven timeline could deliver a vaccine in as little as 18 months, most vaccines take years, in fact often more than 10 years, to go from preclinical testing to a syringe in the doctor’s office. a doctor.
Reducing that timeline can increase the risk of failure. For example, vaccine candidates generally enter all three phases of clinical trials only after they have been well tested in laboratory animals that can model human disease. But, with such a new virus, there is no established animal model for COVID-19. And in the midst of a devastating pandemic, there is not enough time to fully develop it. Some researchers are now doing that work with animals at ground level in parallel with the human trials, like the small monkey trials mentioned above.
The researchers already have reason to be a little anxious about the safety of any COVID-19 vaccine. When they tried in the past to make vaccines against some of the relatives of the SARS-CoV-2 coronavirus, they found a small number of cases in which the candidate vaccines seemed to worsen infections. That is, these candidate vaccines appeared to elicit frenetic immune responses that caused lung damage in monkeys and liver damage in ferrets. The researchers still don’t fully understand the problem and don’t know if it could happen in humans, let alone if it will appear with the new candidate vaccines against SARS-CoV-2.
But we will soon know the answers. As the pandemic surpasses the grim milestone of three million cases worldwide and more than 200,000 deaths, researchers relentlessly move forward with vaccine development. This is where the scientific community is currently in its frantic effort.
First, the basics
Researchers are using a wide variety of tools and techniques to develop a vaccine: some are tried and tested, others are new and untested. Regardless of strategy, they all aim to do the same thing: train the immune system to identify SARS-CoV-2 (or some element of it) and destroy it before it establishes an infection and causes COVID-19.
The way a vaccine can accomplish this is generally by feeding immune cells a characteristic element of a disease-causing germ, like a unique protein that lines the outside of a dangerous virus. From there, a type of white blood cell called B cells can generate antibodies that specifically recognize and encompass those characteristic germ elements. Antibodies are Y-shaped proteins, which have their germ-specific detection regions in their outstretched arms. The base of its “Y” shape is a generic region that can indicate certain immune responses if they detect an invading germ.
A strong and effective vaccine can generate so-called neutralizing antibodies. These antibodies circulate in the blood, monitoring the entire body after a vaccine is administered. If the germ they are trained to detect actually appears, the antibodies can swarm it and paralyze it. The antibody base, now hanging from its suffocated target germ, can signal immune cells to help finish the job.
In the case of COVID-19, the goal of candidate vaccines is to train our immune system to produce antibodies that specifically detect and destroy SARS-CoV-2 (which is, again, the new coronavirus that causes COVID-19) . Although there are many things we don’t know about SARS-CoV-2, we know the basics of directing early vaccine development.
We know that SARS-CoV-2 is a betacoronavirus related to two other notorious betacoronaviruses: SARS-CoV-1, which causes SARS (severe acute respiratory syndrome), and the Middle East respiratory syndrome coronavirus (MERS-CoV). , which causes MERS.
Coronaviruses generally maintain their genetic blueprints in the form of a large, single-stranded, positive-sense RNA genome that clusters into a round viral particle. That genetic code provides the molecular instructions for making all of the virus’s components, including the enzymes necessary to make copies of the virus’s genome and the virus’s famous spike protein.
Spike protein is what coronaviruses use to get hold of host cells, that is, human cells that infect or the cells of any other animal victim. Once the virus catches on with its spike protein, it enters the cell and hijacks the cell’s activities, forcing it to help make viral clones, which then explode to infect more cells.
There are many copies of the spike protein on the outer surface of coronaviruses, creating a pointy exterior – think of a cartoon marine mine. Pointy ornaments are actually what give coronaviruses their name. Under an electron microscope, the spikes give the viral particle a corona appearance, therefore crown Viruses But most importantly, spike proteins are a prime target for antibodies. And since we have the entire genome sequence for SARS-CoV-2, the researchers are off to a good start in discovering effective ways to design vaccines to attack spike proteins and other critical components of the virus.
Vaccination platforms
There are many ways to try to train the immune system to fight a specific germ or specific elements of germs, such as SARS-CoV-2 or the spike proteins of SARS-CoV-2. Here are the general categories currently at stake:
Live attenuated vaccine: These vaccines use whole viruses that are weakened so they can no longer cause disease. This is a well established method of creating vaccines. In the past, researchers weakened viruses by growing them under laboratory conditions for long periods of time, which is a bit like taming germs. The comfortable and complete lifestyle of the Petri dish can essentially allow viruses and bacteria to adapt to their calm environment and lose virulence over time. But, it may take a while. Scientists developed the measles virus under laboratory conditions for almost 10 years before using it for a live, attenuated vaccine in the early 1960s.
Today, there are faster, more controlled approaches to designing weakened viruses, such as specific mutations and other manipulations of a virus’ genetic code.
Live attenuated virus vaccines have the advantage of generating the same variety of protective antibodies as a real infection, without causing, for the most part, annoying and life-threatening disease. But there are risks. Because the virus can still replicate, certain people (particularly those with immunodeficiencies) can have severe reactions. Although newer strategies to weaken viruses can reduce these risks, they still require extensive security testing before they hit the market.
That being said, this is a vaccine platform that has already proven to be successful. Several vaccines in use are live attenuated vaccines, including vaccines against chickenpox and typhoid fever. If such a vaccine was effective in preventing COVID-19, we already have the knowledge and infrastructure to rapidly expand production to produce these vaccines.
Inactivated vaccine: This is another simple and traditional method that uses complete viruses. However, in this case, the viruses are effectively dead, generally inactivated by heat or chemicals. These cadaveric viruses can still prime the immune system to produce neutralizing antibodies; they just do it less efficiently.
The advantage of this strategy is that it is relatively easy to manufacture these types of vaccines and, because viruses do not replicate, there is no risk of infection and less risk of serious reactions. Disadvantages include that inactivated viruses that do not replicate do not produce an immune response as strong as a debilitating or disease-causing virus. Inactivated vaccines always require multiple doses and may also require periodic booster injections.
Like vaccines with weakened viruses, the use of a complete viral particle gives the immune system many potential viral targets for the antibodies. Some may be good targets for neutralizing a real infection, and others may not. But, using an inactivated virus is a proven method. For example, some existing polio, hepatitis A, and rabies vaccines use this method.
Vector-based viral vaccine: For these vaccines, researchers take a weakened or harmless virus and design it to contain an element of a dangerous virus they want to protect themselves from.
In the context of COVID-19, this could mean designing a harmless virus to produce, for example, the spike protein of SARS-CoV-2. In this way, you get the immune response to a live but benign virus, along with the probability of having antibodies that target a specific critical protein of the dangerous SARS-CoV-2.
This is also a proven strategy for effective vaccines. The recently approved Ebola vaccine, for example, uses this method.
Vaccine subunits: These are basic vaccines that include only one component of a dangerous virus to provoke immune responses. For COVID-19 vaccines, the spike protein is, not surprisingly, a popular candidate.
The subunits can be administered in formulations with adjuvants, accessory ingredients that can enhance immune responses. A common adjuvant is alum, an aluminum salt, long known for being useful in vaccines. However, some newer subunit vaccines come in faster packages. These include artificial “virus-like particles” (VLPs) and nanoparticles.
Subunit vaccines are already an established vaccine platform. The HPV vaccine in use involves a VLP that feeds proteins from the immune system from the outer covering of HPV, which can then be attacked by antibodies.
RNA and DNA vaccines: These are among the newest types of vaccines, and among the most unstable. There are currently no licensed vaccines using this method. But researchers are optimistic about its potential.
The basic idea is to deliver genetic material from a virus, either in the form of DNA or RNA, directly to human cells, which are then forced to translate that genetic code into viral proteins and can then produce antibodies against them.
Some of the details of how these candidate vaccines work are patented and unproven, making it difficult to assess how likely they are to be successful or how easy it will be to increase vaccine production if they are successful.