[ad_1]
Betelgeuse is an incredibly large star located about 700 light-years away from Earth. It is approximately 800 times wider than the Sun and almost 15 times larger. It is also the second brightest star (fig. 1) after Rigel in the Orion constellation, in the northern hemisphere.
Recently, Betelgeuse’s brightness began to dim in an unprecedented way, attracting the attention of astronomers and amateur astronomers from around the world. The brightness of most stars, including our Sun, varies with time. But these variations are usually small, nothing more than a few percentage points of the star’s total light output. Betelgeuse, however, has been doing something else.
It started to fade sometime in October 2019 and had lost two-thirds of its brightness in mid-February 2020. Betelgeuse, which was normally ranked as the 10th brightest star in the night sky, suddenly dropped to 25. This is one Impressive drop that we haven’t noticed with any other stars before, and many astronomers and astrophysicists have been struggling to make sense of the stellar drama.
Some astronomers suspect that the star is approaching its death. They argue that Betelgeuse’s bizarre decline could soon culminate in a sudden end brought on by a violent explosion known as a supernova. However, given how old and close we know Betelgeuse to be, a supernova event in our lives seems quite unlikely.
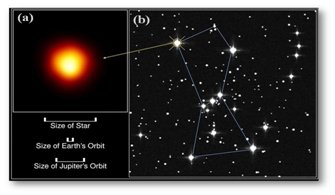
Betelgeuse belongs to a category of massive stars that are extremely rare. There are more low-mass stars in the Milky Way galaxy than there are high-mass stars. Astronomical studies of the night sky have found that the star count drops significantly as mass increases. On average, for every 200 stars, there is only one Betelgeuse-type star.
So calling Betelgeuse supergiant would not be an exaggeration. It is so big that 800 million soles could fit inside it, and each Sun can pack in 1.3 million Earths.
The luminosity of a star is the amount of energy released from its surface every second. Betelgeuse’s luminosity is 100,000 times that of the Sun. However, its surface is also colder, 3,600 K compared to 5,800 K from the Sun, so only about 13% of its radiant energy is emitted as visible light.
Traditionally, Betelgeuse is classified as a pulsating variable star. This means that the brightness of the star changes as the star expands and contracts. In the past, Betelgeuse has shown striking and unambiguous pulsation phases. Corresponding changes in brightness were first noted by English astronomer John Herschel in 1836. In the past two centuries, the star is reported to have undergone several intermittent phases of brightness and dimming.
In 1920, Betelgeuse became the first star to measure its angular diameter with a technique called interferometry, by Albert A. Michelson2 and Francis Pease.
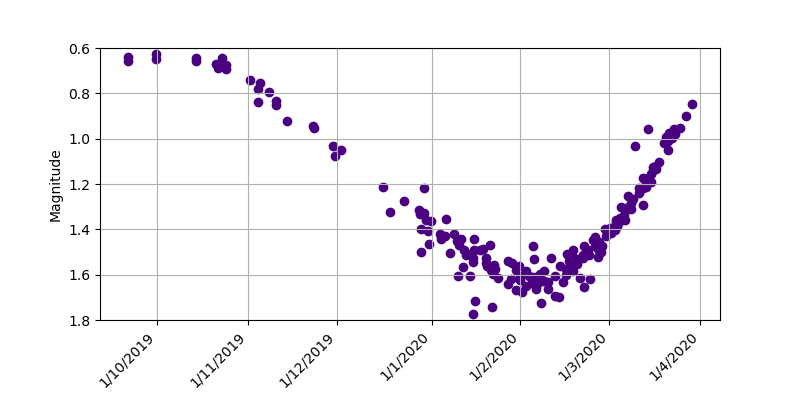
What happens inside a massive star?
Each star constantly has to contend with two competing sets of forces throughout its life: the force of gravity that holds the star together and the forces that drive nuclear reactions that are the star’s energy source. Stars are primarily cosmic factories that fuse lighter elements into heavier elements. The star’s gravity pulls everything inward, while the heat and radiation from the reactions exert external pressure. The balance of these two opposing forces holds the star together. Think about how a pressure cooker works. The hot steam inside the kitchen is like the energy from nuclear reactions. More heat creates more pressure and the steam tries to escape by forcing the lid open. The weight of the cap, or whistle, is like gravity: it keeps the pressure under control.
The mass of a star generally ranges from 0.1 to 150 times the solar mass. A “normal” star like the Sun burns its fuel slowly and lives for several billion years, while massive stars like Betelgeuse have a short life, on the order of millions of years, because they consume their nuclear fuel faster.
Also read: WTF: The story of the strangest star we’ve ever met
Supergiant stars produce heavier elements like iron inside them in a series of nuclear combustion cycles. The time scale of the different stages of combustion is determined by the initial mass of the star. Each star spends about 90% of its lifespan fusing hydrogen into helium within the core. Subsequently, helium was fused into carbon, carbon into neon, neon into oxygen, etc. In high-mass stars, iron is the end product of this series of fusion reactions. And since iron’s atomic nuclei are very stable and tightly bonded, they can’t fuse together anymore. Nuclear reactions then stop when the core of the star is filled with iron.
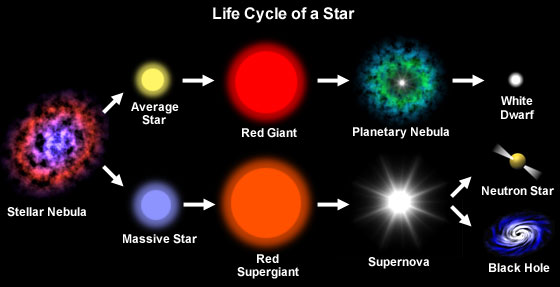
Without nuclear fuel, the core begins to cool even when there is nothing to push against the force of gravity, so gravity takes the lead. In less than a second, the iron core collapses catastrophically, forcing the material on the outer parts of the star to fall freely into the shrinking core. The falling matter hits the heated core with tremendous force and bounces violently in the form of a shock wave traveling into space. This process produces heavier metals like gold and platinum, as well as gravitational waves and fast neutrinos. The amount of energy released by such powerful explosions can momentarily exceed the combined energy of all the stars in the host galaxy.
After this cataclysm, what remains of the nucleus becomes a neutron star or, if it is dense enough, a black hole.
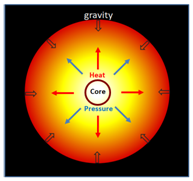
Betelgeuse is already around 10 million years old, and is the most promising star in the night sky to become a supernova in the future. We can only speculate on Betelgeuse’s fate and cross our fingers in hope. There is no exact way to predict the exact moment of his disappearance. That said, when it turns into a supernova, instruments on Earth will record gravitational waves and fast neutrinos from the explosion several hours before visual fireworks appear. This is because gravitational waves are generated just before the explosion, travel at the speed of light, and are not disturbed by the intervening matter. Neutrinos also travel at almost the speed of light and don’t interact much with matter.
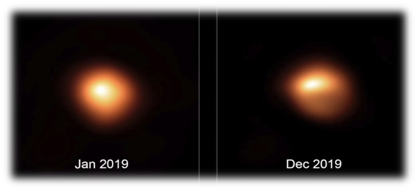
Plausible explanations
Point hypothesis
The energy produced in the center of the star has to leave and reach the surface. In high-mass stars, energy is carried by large droplets of hot, ionized material that rises to the surface, much like bubbles rising from the bottom of a pot of boiling water over a stove. These superheated drops of plasma are called convective cells. In a Sun-like star, convective cells are only a few hundred kilometers wide. At Betelgeuse, they are about 240 million kilometers wide, the entire distance between Earth and Mars.
In fact, the surface of most stars is occasionally joined by strong magnetic fields called star points (just like sunspots). The magnetic field at these points prevents the energy inside the star from transforming at the surface. Therefore, the point regions are cooler and emit less energy. And yes, the bigger the star, the bigger the spots.
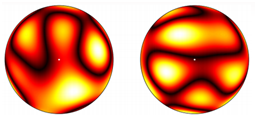
A giant dot covering the Betelgeuse surface may have temporarily prevented convection over a large area, reducing the surface temperature of the supergiant. This would explain the current attenuation.
However, astronomers Emily Levesque and Philip Massey have discovered in more recent observations at the Lowell Observatory, Arizona, that Betelgeuse is not that great after all. In a scientific article that appeared in the March 2020 issue of the Astrophysical Journal, they reported a measured temperature not unlike what previous studies have found, meaning the star hasn’t undergone the kind of substantial cooling that could explain its brightness deficit.
Therefore, the point hypothesis is unlikely to be the main cause of attenuation.
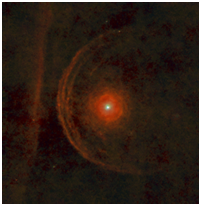
Powder hypothesis
At the last stage of its evolution, each star is known to lose mass. Although Betelgeuse is huge, it is 117.5 million times less dense than the Sun, which means it has low surface gravity and a small escape velocity: 60 km / s versus 600 km / s from the Sun. This in turn it means that gas and dust escape more easily from the Betelgeuse surface into the circumstellar medium3. And in this way, Betelgeuse has been losing a mass of material from Earth every year, material that condenses to form a nebula-like envelope of gas and dust that can be seen in images taken at infrared wavelengths.
Some astronomers think that an oddly shaped column of dust and gas produced in this way has simply gotten in our line of sight and prevented some of the starlight from Betelgeuse from reaching Earth.
This fortuitous conjunction appears to have lasted until mid-February 2020. These days, the star appears to be regaining its lost glow.
The dust hypothesis seems to offer a satisfactory explanation of the attenuation. However, we still need further observations to confirm this possibility beyond any reasonable doubt.
§
All the stars die. The older ones simply die more spectacularly.
Betelgeuse is too far from Earth to pose a major threat when it finally explodes, but it is close enough to offer a unique opportunity for astronomers and astrophysicists to study the rare cosmic event in great detail. Your supernova will be brighter than the full moon at night and will be visible even during the day.
Betelgeuse also has a life too short (in stellar terms) for planets to form around it, leaving only port life. However, Betelgeuse and her supergiant peers are progenitors of life in a different way. The heaviest elements formed in the core of a massive star are ejected into the interstellar medium after the supernova. These wastes mix with gas and dust to become the material for the subsequent generation of Sun-like stars, which then support planets.
Also read: The history of dust, through space and time
In fact, we owe our existence to the death of a massive star. Our Solar System was formed from the remains of a similar explosion that preceded the birth of the Sun. Many essential ingredients of the human body were first created in a distant supernova. In the grand scheme of things, we are truly children of stardust, and this is possibly the most profound and humiliating thing that modern science has helped us find.
Ravinder Banyal is a research scientist at the Indian Institute of Astrophysics, Bengaluru.